Vivianite
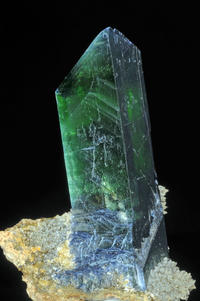
a vivianite specimen from Huanuni Mine, Huanuni, Dalence Province, Oruro Department, Bolivia
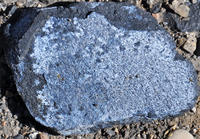
Vivianite weathering crust on phosphorite (Meade Peak Member, Phosphoria Formation, Permian; Astoria Hot Springs roadcut, Teton County, Wyoming, USA
Mineralogical Data
Chemical Formula:
Fe2+3(PO4)2 · 8H2O
Crystal System:
Monoclinic
Morphology:
Prismatic crystals, reniform or globular aggregates, tabular masses or concretions, crusts with fibrous to bladed structure, and earthy coatings
Appearance:
Colourless and transparent when fresh, quickly turning a transulcent pale green/blue to deep blue
Moh's Hardness:
1.5-2
Density:
2.67 - 2.69 g/cm3 (Measured)
2.696 g/cm3 (Calculated)
Tenacity:
Sectile
Other Names:
anglarite
mullicite
Related Links
Vivianite is a hydrated iron phosphate mineral, which has historically been used as a blue pigment in wall (Howard 1996) and canvas paintings, as well as on polychrome objects (Scott and Eggert 2007). It also occurs as a blue alteration product on archaeological material in burial environments with a high phosphate concentration (Frost et al. 2003, Scott and Eggert 2007).
Vivianite is known for its rapid colour change, altering from colourless to pale green or blue to a deep blue upon exposure to air (Watson 1918). This change is caused by the oxidation of iron by atmospheric oxygen.
These three photos display the variation in colour that occurs during the partial oxidation of vivianite.
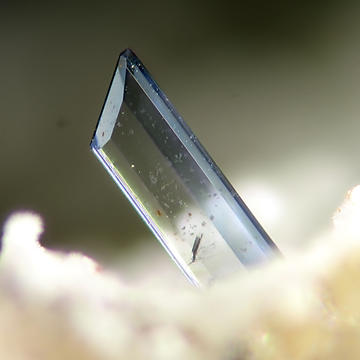
a transparent, pale blue crystal of vivianite from Hagendorf South Pegmatite (Cornelia Mine; Hagendorf South Open Cut), Hagendorf, Waidhaus, Vohenstrauß, Oberpfälzer Wald, Upper Palatinate, Bavaria, Germany
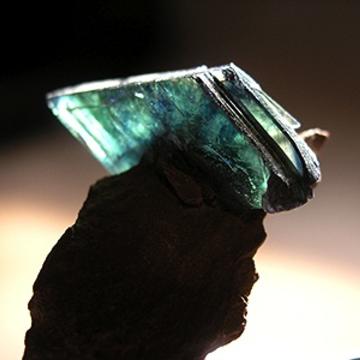
A transparent, blue-green crystal of vivianite; 2 cm doubly-terminated. The mineral displays a darker blue about the centre where it's attached to the matrix. Specimen from Tomokoni mine, Machacamarca District (Colavi District), Cornelio Saavedra Province, Potosí Department, Bolivia
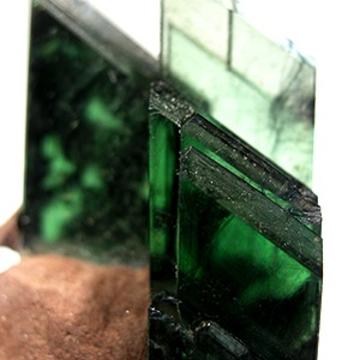
Green crystals of vivianite (4.1 x 2.4 x 1.4 cm). Most notable is the variation in translucency. Two are an opaque dark green with translucent areas of emerald green. Whereas the rearmost crystal is a transparent pale green. Specimen from Tomokoni mine, Machacamarca District (Colavi District), Cornelio Saavedra Province, Potosí Department, Bolivia.
Oxidation
When fresh surfaces of vivianite are exposed to atmospheric oxygen, Fe2+ is oxidised to Fe3+. To satisfy charge requirements, protons are cleaved from surrounding water ligands, resulting in the formation of hydroxyl (OH-) ions (Moore 1971, McCammon and Burns 1980).
2[Fe2+–H2O] + ½O2 => 2[Fe3+–OH-] + H2O
The reaction severely alters the mineral structure, induces further oxidation (by exposing unoxidized Fe), and ultimately results in structural collapse (McCammon and Burns 1980). This is because hydrogen bonds are necessary for vivianite to be structurally stable (Moore 1971, McCammon and Burns 1980). The conversion of H2O to OH- results in a reduced capability for hydrogen bonding between octahedra, affecting intrachain connectivity. This can result in a flaky appearance (McCammon and Burns 1980), as unbound chains can easily exfoliate from the mineral surface.
As with any oxidation reaction, the rate of alteration is limited by the permeability of oxygen through the oxidation product and the availability of fresh surfaces.
Dehydration
Vivianite surfaces have been observed to be relatively stable to oxidation compared to the bulk crystal. This has been attributed to surficial dehydration forming a “more stable lower hydrate of formula Fe3(PO4)2·nH2O where n <8” (Tricker et al. 1979: 892).
It is difficult to say whether vivianite can dehydrate without (first) oxidising. ‘Dehydration’ is part of the oxidation process, as water is released as a reaction product. Yet, the extent of dehydration necessary to form a stable product is much greater than the ~1H2O per formula unit attributed to the oxidation process.
Two known lower hydrates of iron(II) phosphate are ludlamite (n=4) and phosphoferrite (n=3). Both also oxidise rapidly upon exposure to air, with ludlamite becoming structurally unstable. Oxidised phosphoferrite, on the other hand, is structurally stable, and will appear dark green to blue in colour like vivianite (Moore 1971).
This extent of dehydration—the loss of 5 molecules of H2O per formula unit—has been documented. Thermal analysis has shown that dehydration begins at or around room temperature (Tien and Waugh 1969, Marincea et al. 1997), absorbed water is lost by 50°C, and that 5 moles of water are lost by 105°C (Frost et al. 2003).
Even though this water loss is documented at elevated temperatures, it is possible that vivianite could dehydrate in a museum environment over time if the right conditions present themselves. In turn, it could theoretically be possible for vivianite crystals to have a thin, visually unobtrusive layer of phosphoferrite on exposed surfaces. However, further evidence, such as XRD analyses, is necessary to confirm this.
Colour
Vivianite’s colouration is related to iron oxidation. Wherry addressed the colour change as a note in Watson 1918; “Since ferrous iron usually colors minerals green, and ferric iron yellow or brown, it may seem rather remarkable that the presence of both together should give rise to a blue color,” (ibid. 161). Thus, it can be assumed that green specimens contain less Fe3+ than blue specimens. Likewise, the intensity of blue in specimens can be related to Fe3+ content: “strongly colored varieties of the mineral invariably show the presence of ferric oxide in quantity ranging up to 33 per cent. or more” (ibid. 159). The ‘extensively oxidised’ samples from McCammon and Burns' (1980) study were dark blue and >35% oxidise.
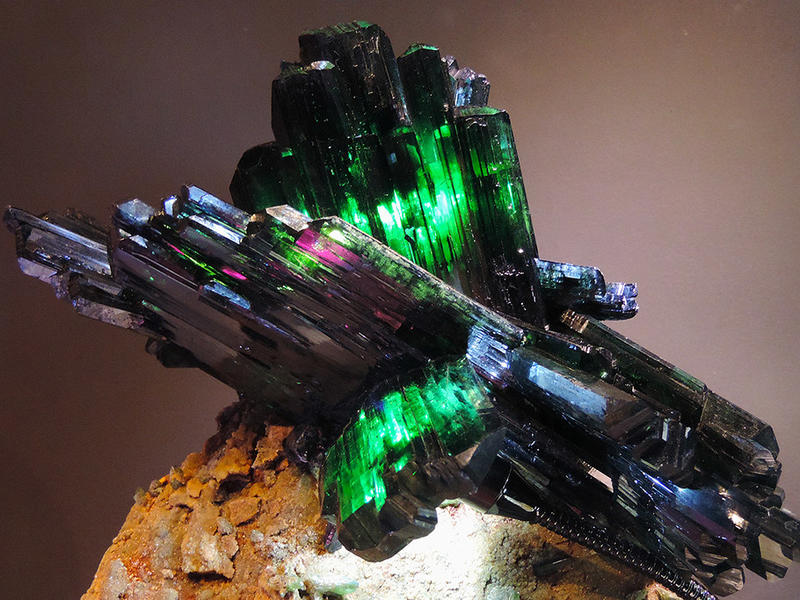
a very colourful specimen of vivianite from The Perot Museum of Nature and Science (Texas, USA)
Recommendations
Most specimens in collections are partially oxidised (Marincea et al. 1997), according to formula:
Fe2+3-xFe3+x (PO4)2(OH)x · (8 -x)H2O, where x<1.2
Most of oxidation occurred when the specimen was first exposed to air. The initial oxidation is quite rapid, but the reaction plateaus, as subsequent oxidation is limited by the diffusion of oxygen into the crystal structure and limited availability of fresh surfaces.
It is impossible to halt vivianite's oxidation altogether. But it is possible to avoid additional periods of rapid, extensive oxidation by mitigating the creation of fresh surfaces. This can be done by minimising handling, using clean gloves and working surfaces to mitigate abrasion, and ensuring the mineral is well-supported at all times to minimise the risks of breakages.
One may think that the use of anoxic environments or coatings could be used to halt vivianite's oxidation, but the benefits are negible at best. Oxygen from absorbed or condensed water could still promote oxidation within an anoxic environment that does not exclude moisture. And coatings could react with the mineral, altering its appearance and lustre. Both also inhibit scientific analysis, albeit in different ways.
Additionally, vivianite should not be exposed to high temperatures. The rate of oxidation and dehydration increases with increaing temperature (Tien and Waugh 1969, Marincea et al. 1997, Frost et al. 2003, Čermáková et al. 2015). Temperature-induced oxidation begins at 90°C, although temperatures greater than 70°C have been found to be determinatal to vivianite's appearance and structure (Čermáková et al. 2015). Thus, vivianite should not be illuminated by heat-generating light sources, and any conservation treatments of artworks containing vivianite as a pigment should be performed at temperatures below 70°C to avoid colour alteration.
References:
-
Čermáková, Z., Švarcová, S., Hradilová, J., Bezdička, P., Lančok, A., Vašutová, V., Blažek, J., and Hradil, D., 2015. Temperature-related degradation and colour changes of historic paintings containing vivianite. Spectrochimica Acta - Part A: Molecular and Biomolecular Spectroscopy, 140, 101–110.
-
Frost, R.L., Weier, M.L., Martens, W., Kloprogge, J.T., and Ding, Z., 2003. Dehydration of synthetic and natural vivianite. Thermochimica Acta, 401 (2), 121–130.
-
Howard, H., 1996. Romanesque and Gothic wall paintings in Winchester Cathedral: an unusual use and alteration of vivianite. Journal of the Russel Society, 6 (2), 93–96.
-
Marincea, S., Constantinescu, E., and Ladriere, J., 1997. Relatively unoxidized vivianite in limnic coal from Capeni, Baraolt Basin, Romania. Canadian Mineralogist, 35 (3), 713–722.
-
McCammon, C.A. and Burns, R.G., 1980. The oxidation mechanism of vivianite as studied by Mossbauer spectroscopy. American Mineralogist, 65 (3–4), 361–366.
-
Moore, P.B., 1971. The Fe2+3(H2O)n(PO4)2 homologous series: crystal-chemical relationships and oxidized equivalents. American Mineralogist, 56 (1–2), 1–17.
-
Scott, D.A. and Eggert, G., 2007. The vicissitudes of vivianite as pigment and corrosion product. Studies in Conservation, 52 (sup1), 3–13.
-
Tien, P.-L. and Waugh, T.C., 1969. Thermal and X-ray studies on earthy vivianite in graneros shale (Upper Cretaceous), Kansas. American Mineralogist, 54 (9–10), 1355–1362.
-
Tricker, M.J., Ash, L.A., and Jones, W., 1979. On the anomalous inertness to oxidation of the surface regions of vivianite: A 57Fe conversion electron and transmission Mossbauer study. Journal of Inorganic and Nuclear Chemistry, 41 (6), 891–893.
-
Watson, T.L., 1918. The color change in vivianite and its effect on the optical properties. American Mineralogist, 3 (8), 159–161.