Tin
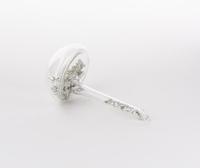
A mushroom-shaped glass vial containing tin, from the Science Museum Group, object number 1977-903/41
"Mushroom shaped glass vial containing tin (element)" by the Science Museum Group
Mineralogical Data
Chemical Formula:
Sn
Crystal System:
tetragonal
Morphology:
grains, nuggets
Appearance:
metallic silvery, white
Moh's Hardness:
1.5-2
Density:
7.31 g/cm3 (Measured)
7.286 g/cm3 (Calculated)
Tenacity:
maleable
Related Links
Tin is a silvery grey metallic element. It is #50 on the periodic table, belonging to period 5, and group 14. Its abbreviation, Sn, comes from the Latin word stannum.
An Ancient Metal
Tin is one of the seven ‘metals of antiquity’ (Challoner 2019), along with gold, silver, copper, lead, iron, and mercury. In alchemy, each of these metals was associated with one of the seven classical planets (a.k.a. the ‘wandering stars’) and their respective deities.
Tin has had a variety of uses throughout history, including as a solder, plating, and alloying material (Challoner 2019). Two common tin-containing alloys are bronze and pewter. Both are ancient alloys and have been produced since the Bronze Age. Bronze production began in the ancient Near East c. 3300 BCE, although tinned bronzes have recently been excavated in Serbia (Radivojevic et al. 2013) which pre-date Near Eastern bronzes by c. 1500 years. Pewter production also began in the Near East; the oldest pewter object was found in an Ancient Egyptian tomb, and dates to c. 1450 BCE (Hull 1992). However, it is likely that pewter had been in use for some time prior to then.
Metal |
Abbreviation |
‘Planet’ |
Symbol |
Gold |
Au |
Sun |
⊙ or ☼ |
Silver |
Ag |
Moon |
☽ |
Mercury |
Hg |
Mercury |
☿ |
Copper |
Cu |
Venus |
♀ |
Iron |
Fe |
Mars |
♂ |
Tin |
Sn |
Jupiter |
♃ |
Lead |
Pb |
Saturn |
♄ |
Material Properties
Tin has two allotropes, α and β. An allotrope is “the property of some chemical elements to exist in two or more different forms [whilst] in the same physical state” (Wikipedia 2021). The properties of tin’s two allotropes differ greatly, and the transition from one allotrope to the other can have some dramatic effects.
Allotrope |
α-Tin |
β-Tin |
---|---|---|
|
Non-metal, |
Metal |
Colour |
Grey |
White |
Lustre |
Dull |
Silvery, metallic |
Tenacity |
Brittle |
Malleable |
Crystal Structure |
Cubic |
Tetragonal |
Density |
5.77 g/cm3 |
7.31 g/cm3 |
β → α Transition
The β to α transition in tin has been known since at least the time of Aristotle (c. 350 BCE), and was termed ‘tin pest’ or ‘tin plague’ due to the formation of blisters and warts on the object’s surface (Plumbridge 2007, Di Maio & Hunt 2009, Zeng et al. 2014). Tin pest negatively affected Napoleon’s troops fleeing from Moscow in 1812 and the crew of Scott’s Terra Nova Antarctic expedition in 1912, destroying tin objects and solder, and “leaving the men hungry and cold” (Mitchell & Donnelly 1991: 747).
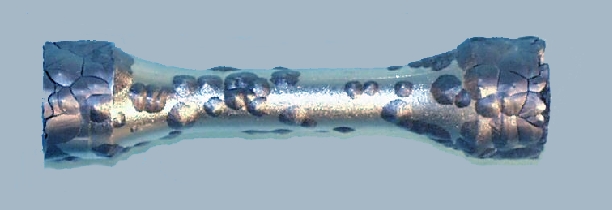
A cast test piece of tin that had developed cracks and warts of tin pest after 1.5 years of exposure to -18°C. A higher concentration of warts is displayed on the machined areas.
The allotropic transition of tin is the only known solid state transition where a metal becomes a non-metal (Vnuk 1980, Zeng et al. 2014). The transition begins when the temperature is below 13.2°C (55.7°F) under atmospheric pressure. However, the transition is often only perceptible after a large degree of undercooling (T < -13°C) and or a considerable incubation period, which can be days or even years (Ojima et al. 1993, Plumbridge 2007, Di Maio & Hunt 2009) depending on the temperature.
The incubation period is when seed crystals of α-tin form (Zeng et al. 2014). This process requires a lot of energy, as bonds have to be broken to allow for the reconfiguration of the crystal lattice. Hence the significant degree of undercooling and length of time required. The incubation period takes longer with increasing tin purity (Mitchell & Donnelly 1991). Conversely, incubation can be reduced by the inclusion of pre-existing α-tin or impurities with a similar, cubic structure (Mitchell & Donnelly 1991, Nogita et al. 2013, Zeng et al. 2014). Upon completing the incubation period, the phase transition occurs more readily and relatively rapidly (Plumbridge 2007, Zeng et al. 2014). Starting at the seed crystal, the α-tin progressively moves into and transforms the surrounding β-tin (Di Maio & Hunt 2009).
Due to the difference in crystal structure, the β → α transition results in a large volumetric expansion (~27%) that produces great stress within the sample. Stress is released through cracking, blistering, fragmentation (Vnuk 1980, Ojima et al. 1993), rupture, and the formation of defects, such as twinning and dislocations (Mitchell & Donnelly 1991, Matvienko & Sidelnikov 1997, Plumbridge 2007). The transformation also affects the tenacity of the product α-tin; the presence of internal stresses, microcracks, and defects cause α-tin to be mechanically very weak and brittle (Vnuk 1980), often resulting in its disintegration (Plumbridge 2007, Di Maio & Hunt 2009, Zeng et al. 2014).
Time lapse video by wwwperiodictableru of a β-tin sample maintained at -40°C. One second of the video corresponds to one hour in real time. Grid size is 1 cm2.
Time lapse video by wwwperiodictableru of a circular β-tin sample, seeding in the centre.
The maximum rate of transition for pure tin occurs at -40°C (Plumbridge 2007). As the temperature increases, the transition rate decreases; the rate at -18°C is only 1/3 that at -40°C (Plumbridge 2007). This is because the difference in energy between α- and β-tin becomes smaller as the temperature increases, and the transition can only occur when the “free energy of the product phase [α-tin] is lower than that of the parent phase [β-tin]” (Massalski 1976: 120).
It is this difference in energy between the two phases that drives the transformation (Massalski 1976, Mitchell & Donnelly 1991, Matvienko & Sidelnikov 1997). Part of the energy is used during the reconfiguration of the tin crystal lattice, while the rest is used to move the α/β interface forward (Massalski 1976, Matvienko & Sidelnikov 1997). The rate of the interface’s migration is dependent on the ambient temperature, the presence of any inclusions or impurities, and the shape, size, and thickness of the specimen (Matvienko & Sidelnikov 1997, Zeng et al. 2014). Additionally, the releasing of stress by defect formation or cracking allows for faster interface migration (Plumbridge 2007). This is because the cracks provides free surfaces that enable further transformation (Di Maio & Hunt 2009, Nogita et al. 2013).
Elements soluble in tin can supress and even prevent the β → α transition, whilst elements insoluble in tin promote and accelerate the transformation (Plumbridge 2007, Zeng et al. 2014). Even concentrations as low as 0.1 wt% of an element are capable of significantly affecting the transition rate (Zeng et al. 2014).
Soluble Elements |
Insoluble Elements |
Antimony |
Aluminium |
Cadmium |
Arsenic |
Copper |
Magnesium |
Germanium |
Manganese |
Gold |
Zinc |
Lead |
|
Silicon |
|
Silver |
|
α → β Transition
The reverse (α→β) transition begins when T > 13.2°C, yet is again often only perceptible once T > ~30°C (Vnuk 1980, Mitchell & Donnelly 1991, Plumbridge 2007). This is when the colour changes from grey to white (Ojima et al. 1993). As with the β→α transition, the large degree of superheating needed for the α→β transformation is due to the “large amount of strain energy involved” (Vnuk 1980: 488) in reconfiguring the tin crystal structure. However, once in motion, the transition itself is very rapid (Vnuk 1980, Mitchell & Donnelly 1991).
Vnuk (1980: 488) remarked that α- and β-tin “can coexist almost indefinitely” at temperatures between 0 and 60°C. Samples left under ambient indoor conditions for two years showed no discernible difference between the two allotropes, other than the extent of oxidation, which appeared more marked on the α-tin samples. Thus it is entirely feasible to retain both allotropes of tin within a museum environment.
Recommendations
To prevent tin pest, avoid storing tin below 13.2°C for prolonged periods of time, especially if the specimen or object is pure tin or contains any tin-insoluble elements (listed above) in quantities > 0.1 wt%. What constitutes a ‘prolonged period of time’ depends on the temperature; the colder the temperature, the less time is needed for the β→α transition to occur, and vice versa. If the temperature dips below freezing for one night, your item is likely fine. But if it is kept below freezing for a week or more, there is a chance that tin pest could develop.
If a tin item has been kept below 13.2°C for a length of time you feel uncomfortable with, you can gradually warm the tin up to 50°C (e.g., ~5°C per minute; Nogita et al. 2013), provided that any other materials associated with the item can withstand such heating. Hold the specimen at 50°C for up to an hour before gradually letting it cool to room temperature. This will ensure that any α-tin within the specimen is eradicated and will provide the specimen with greater thermal-stability in the event it is exposed to temperatures below 13.2°C in the future.
There is a caveat to this, however. For mineral specimens of tin, α-tin may have formed naturally within a predominately β-tin specimen prior to collection and accession. Thus heating to eradicate α-tin may compromise its scientific value, and may also potentially affect the structural integrity of the specimen. As with any conservation action, please consider the wider context before proceeding with heat treatment.
On the other hand, tin alloys containing any one of the soluble elements listed above (e.g. pewter) should be temperature-stable even well below freezing, as thermostability requires only 0.1 wt% of an element. However, other composite materials and any previous damage or treatments will affect thermostability.
In general, avoid keeping objects below freezing, in the event the object contains water. The formation of ice will cause damage much sooner than tin pest will.
References:
-
Challoner, J. (2019). The Elements. Andre Deutsch: London. p.116.
-
Di Maio, D., & Hunt, C. (2009). Time-lapse photography of the β-Sn/α-Sn allotropic transformation. Journal of Materials Science: Materials in Electronics, 20(4), 386–391. https://doi.org/10.1007/s10854-008-9739-5
-
Hull, C. (1992). Pewter, Princes Risborough: Shire.
-
Matvienko, A.A., & Sidelnikov, A.A. (1997). The influence of relaxation of stresses occurring during the β→α transformation of tin on the kinetics of the transformation. Journal of Alloys and Compounds, 252(1–2), 172–178. https://doi.org/10.1016/S0925-8388(96)02734-X
-
Mitchell, D.R.G., & Donnelly, S.E. (1991). A transmission electron microscopy study of the β→α-phase transformation of tin. Philosophical Magazine A: Physics of Condensed Matter, Structure, Defects and Mechanical Properties, 63(4), 747–755. https://doi.org/10.1080/01418619108213911
-
Nogita, K., Gourlay, C. M., McDonald, S. D., Suenaga, S., Read, J., Zeng, G., & Gu, Q. F. (2013). XRD study of the kinetics of β ↔ α transformations in tin. Philosophical Magazine, 93(27), 3627–3647. https://doi.org/10.1080/14786435.2013.820381
-
Ojima, K., Taneda, Y., & Takasaki, A. (1993). Direct observation of α → β transformation in tin by transmission electron microscopy. Physica Status Solidi (A), 139(1), 139–144. https://doi.org/10.1002/pssa.2211390111
-
Plumbridge, W.J. (2007). Tin pest issues in lead-free electronic solders. Journal of Materials Science: Materials in Electronics, 18(1–3), 307–318. https://doi.org/10.1007/s10854-006-9025-3
-
Vnuk, F. (1980). Preparation of compact alpha-tin specimens. Journal of Crystal Growth, 48, 486–488. https://doi.org/10.1016/0022-0248(80)90049-4
-
Wikipedia contributors. (2021). Allotropy. Wikipedia, The Free Encyclopedia. [Accessed April 28 2021]
-
Zeng, G., McDonald, S.D., Gu, Q.F., Sweatman, K., & Nogita, K. (2014). Effects of element addition on the β→α transformation in tin. Philosophical Magazine Letters, 94(2), 53–62. https://doi.org/10.1080/09500839.2013.860247